COTS Radiation Assurance
Introduction
For decades, Commercial-off-the-shelf (COTS) electronic components have been an appealing option for space systems due to their advantages in performance, size, weight, and cost when compared to traditional radiation-hardened (radhard) components. However, COTS components come with their own challenges in terms of reliability and radiation performance, demanding careful vetting to ensure mission success while maintaining an acceptable level of failure probability.
Radiation-Hardened Electronics for Space Applications
In space missions, choosing radiation-hardened electronic (EEE) parts has been the norm, governed by stringent quality and reliability standards. Microcircuits from the qualified manufacturers list (QML) undergo thorough screening and qualification processes to ensure acceptable risk levels. QML-V level parts require additional radiation qualification. Manufacturers of "space-grade" radhard components employ isolated wafer fabrication lines with proprietary processes, specifically tailored to minimize radiation-induced vulnerabilities, such as enhanced-low-dose-rate-sensitivity (ELDRS), single-event latchup (SEL), single-event upset (SEU), etc. This radiation-hardened by process (RHBP) approach involves redesigning transistors to reduce radiation-induced charge trapping and improve radiation tolerance. Alternatively, radiation-hardened by design (RHBD) employs modifications to circuit design, like triple mode redundancy to mitigate SEUs, producing EEE components robust against harsh radiation environments.
The Challenges of COTS
While many COTS components demonstrate intrinsic radiation tolerance, they can exhibit significant variability in radiation susceptibility, since there is no lot traceability in the procurement process. This variability can be a significant challenge for radiation assurance and can be difficult to manage. For instance, the cumulative probability of the failure dose for a specific COTS bipolar transistor irradiated with gamma rays illustrates a wide range of failure doses, from 20 to 80 krad(Si), encompassing a variety of mission types in different space environments.
__________
Ref 1. M.A. Xapsos, et al., "Inclusion of radiation environment variability in total dose hardness assurance methodology," IEEE Tran. Nucl. Sci., vol. 64, no. 1, Jan 2017, pp 325 - 331.
Similarly, variability can also impact the response to single-event effects (SEE) and contribute to uncertainties in predicting the on-orbit SEU rate, large differences in the susceptibility to destructive SEE, and more. For example, the manufacturers can make changes to a product’s process fabrication line without notification, thereby completely altering the product’s radiation susceptibility, including the susceptibility to destructive effects such as SEL.
Addressing the Challenge
With the increasing use of COTS components in space missions and the constraints of tight launch schedules and limited resources, addressing these challenges has become a focal point of discussions in the industry. Various approaches exist to manage radiation effects and ensure mission performance and reliability targets are met. Regardless of the approach, it is vital to thoroughly understand the underlying failure or degradation mechanisms when evaluating the suitability of COTS components for space missions. This understanding, coupled with knowledge of the mission's radiation environment, lays the groundwork for parts testing and radiation data analysis.
Leveraging a Radiation Database of COTS, Automotive-grade Parts
To aid the industry in this endeavor, our radiation database comes to the forefront, containing a vast collection of ground test data from COTS and automotive-grade parts. This comprehensive tool allows users to search by part number, part function, SEL LET threshold, TID pass/fail level, DD fluence, and more, offering valuable insights into radiation performance. This easily accessible resource should provide a significant boost for space missions using COTS components. While RadHard technologies remain a foundational platform for the space industry, we also recognize the utility and application of COTS components. The availability of the radiation database empowers mission planners and engineers to make informed decisions in their pursuit of exploration beyond our planet.
Resources:
​
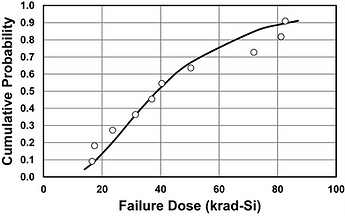
Figure 1. TID failure distribution for the SFT2907A bipolar transistor. The line is a lognormal fit to the data. (After [Ref 1])